🔶 Fate Model of Organic Contaminants in Marine Ecosystems
BACKGROUND
Marine aquaculture farms increase every year due to the increasing demand for seafood worldwide. While providing many benefits, aquaculture systems must be managed carefully to minimize impacts on marine ecosystems.
WHAT WE DO
We are interested in how aquaculture is affecting chemical accumulation and partitioning in aquatic food webs and environmental compartment. We study factors affecting chemical accumulation in water, sediment, air, and fish using field samples and modeling. This provides insights into the effects of marine aquaculture driven changes on chemical exposures and risks.
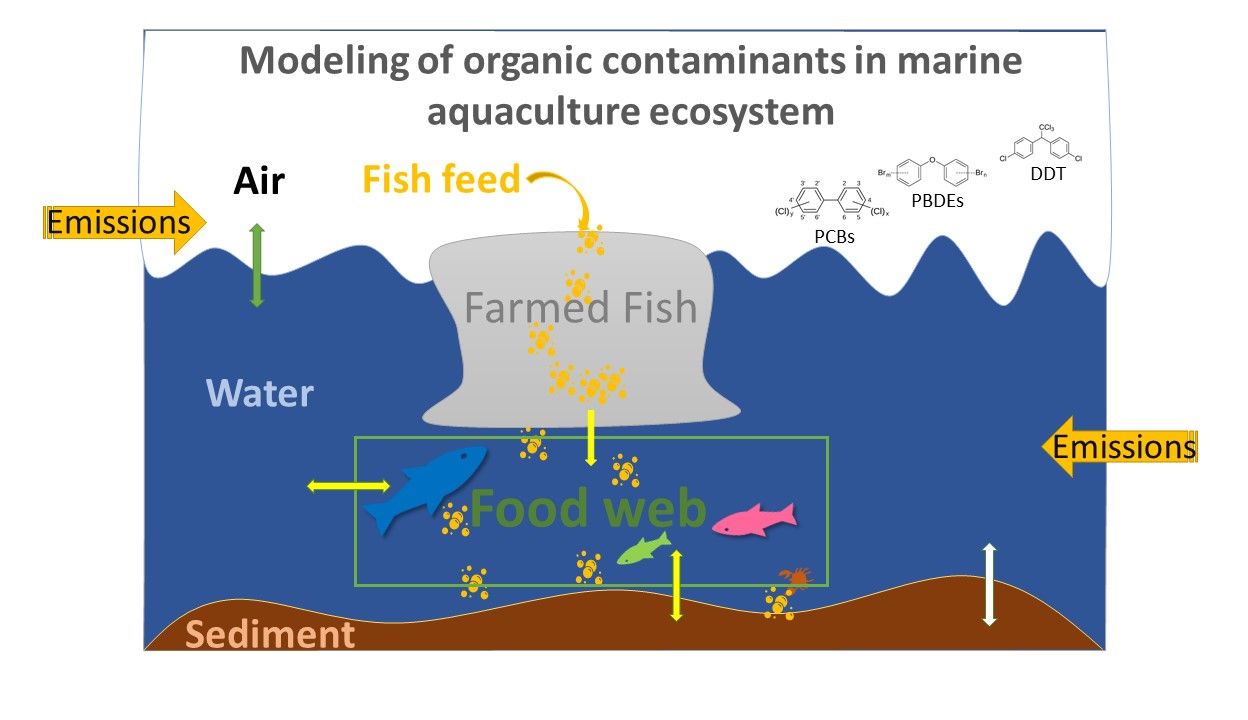
🔷Pesticide Dissipation Model
BACKGROUND
Pesticides have a long history of use in the U.S. and are considered important components of modern farming. However, pesticide volatilization and vapor drift can have adverse effects on nontarget, sensitive ecosystems and human health.
WHAT WE DO
We care about the post-application fate of pesticides in agricultural fields. We have and hope to continue to develop models that allow us to better examine the factors influencing pesticide dissipation/persistence following application.
So far, we have two versions of pesticide model. The first version initially predicted volatilization from soil for the first 24 hours following application. The second version, Pesticide Loss via Volatilization (PLoVo) model, adds a plant compartment and now is capable of predicting the volatilization from plants and/or soil.
The third version, Pesticide Dissipation from Agricultural Land (PeDAL) model, has been successfully developed, including photodegradation on leaf surfaces as well as penetration into leaves. We hope for these models to become tools that farmers use to make better pesticide management decisions and that regulatory agencies use to better protect the health of the environment and those living in it, such as humans and pollinators.

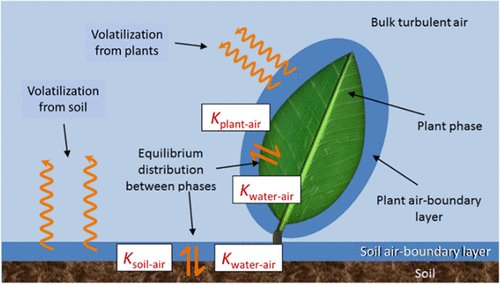
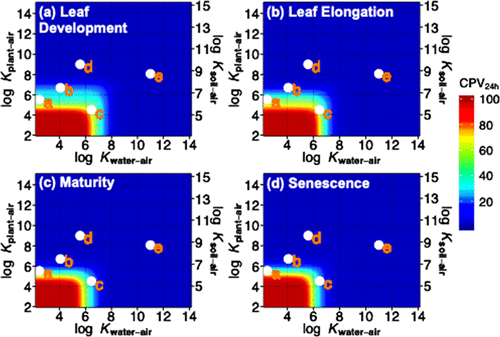
🔶Partition Coefficients of Pesticides
BACKGROUND
Pesticides can either volatilize into the air or deposit onto surfaces in the environment. One property that allows for a prediction of this behavior is the plant-air partition coefficient (Kplant-air). The Kplant-air describes the pesticide's equilibrium that is formed between a plant surface and the air around it after landing on the plant. Differences in temperature, humidity, and the type of leaf influence the Kplant-air, which is why Kplant-air values in the literature are often not applicable for other situations.
WHAT WE DO
We are interested in the relationships between Kplant-air values for different pesticides and different leaves with varying temperature and humidity.
To measure the Kplant-airvalues, a fugacity meter will be used. This pushes gas through a sample chamber containing leaves contaminated with pesticide. As the gas flows over the leaves, the pesticide on the leaf is in equilibrium between the leaf and the air. As the air and volatilized pesticide exit the sample chamber, it flows through a sorbent trap, which retains the gas-phase pesticide. The ratio of the concentration of the pesticide on the plant to the concentration in the air is the Kplant-air.

🔷 Organic Contaminants in Arctic Lakes
BACKGROUND
Many semi-volatile organic contaminants have been shown to travel through our atmosphere far from their point of use. This transport is a result of net volatilization in warmer low-elevation and low-latitude regions and net deposition in colder high-elevation and high latitude regions. As a result, polar regions become global sinks for many organic contaminants, despite their remoteness. While the presence of organic contaminants, especially historic use contaminants, is well documented in the Arctic, the fate of these contaminants thereafter is unknown, particularly for emergent classes of contaminants.
WHAT WE DO
In this project we measure the seasonal trends in the concentrations of emerging brominated flame retardants and current use pesticides in Arctic lake water. Our collaborators study the partitioning and photochemical behavior of these compounds in Arctic lake water. After two summers of field work and laboratory studies, a predictive chemical fate model will be created for an Arctic lake to predict changes in the fate of emergent and current use contaminants in a changing climate.
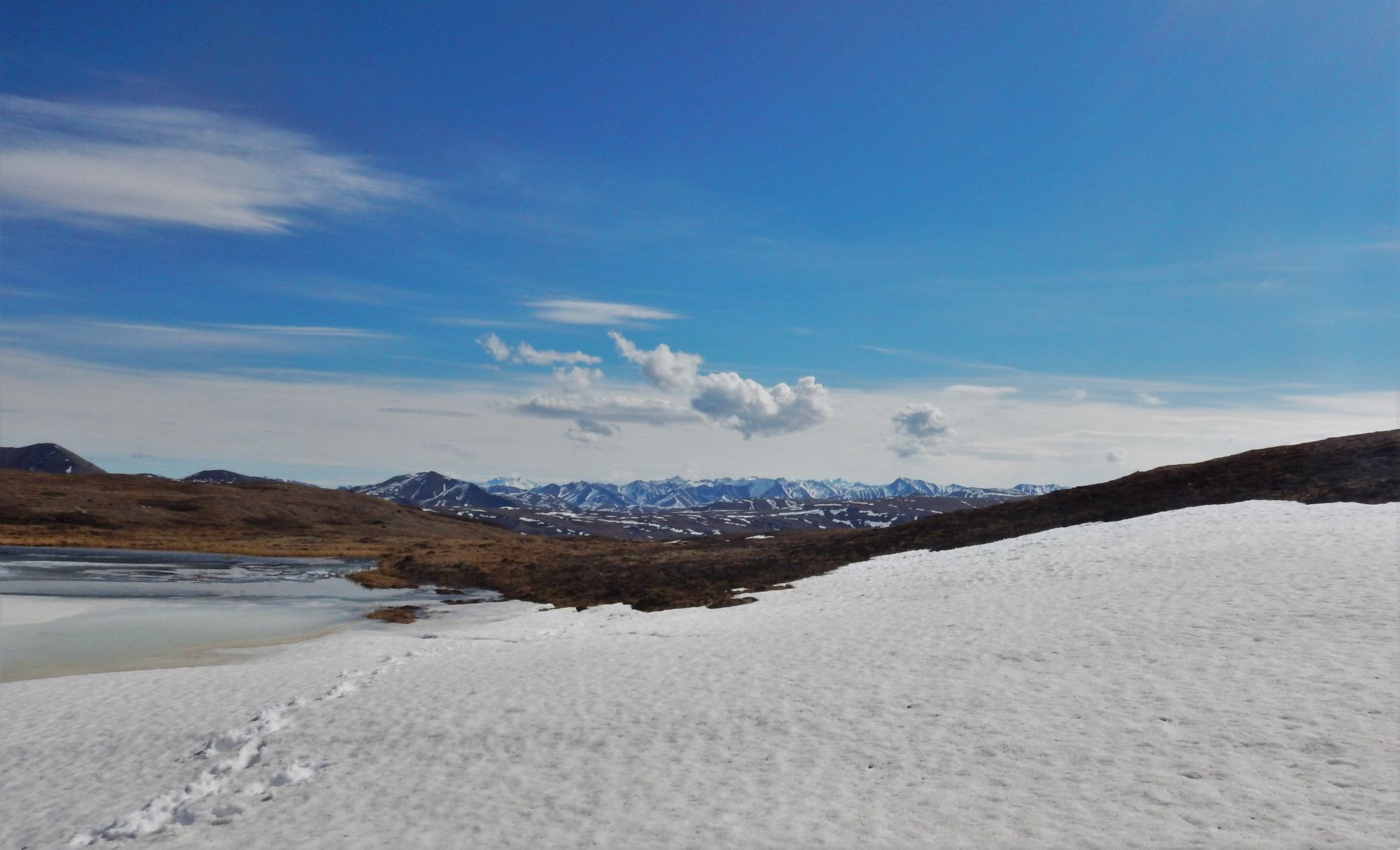
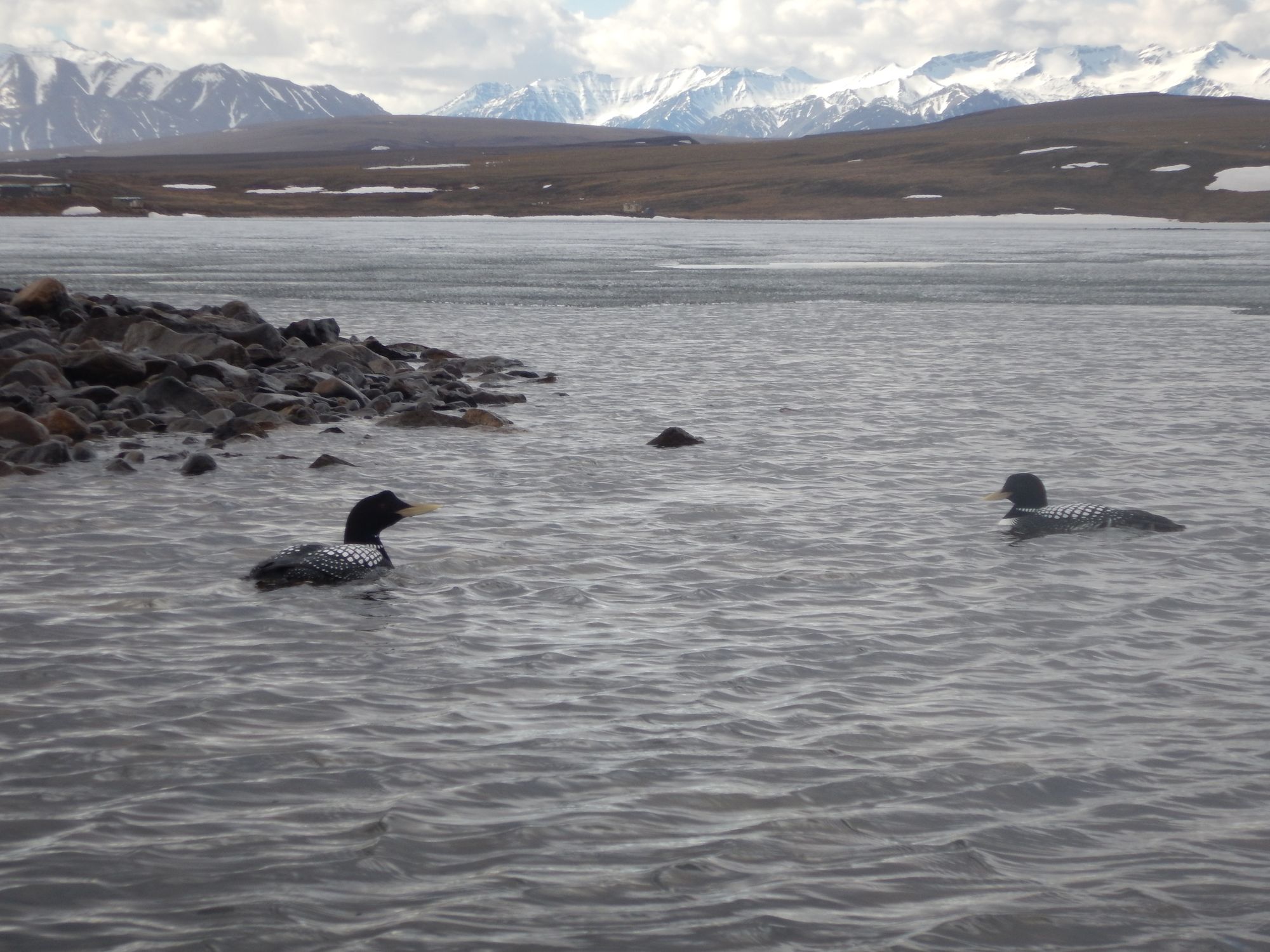
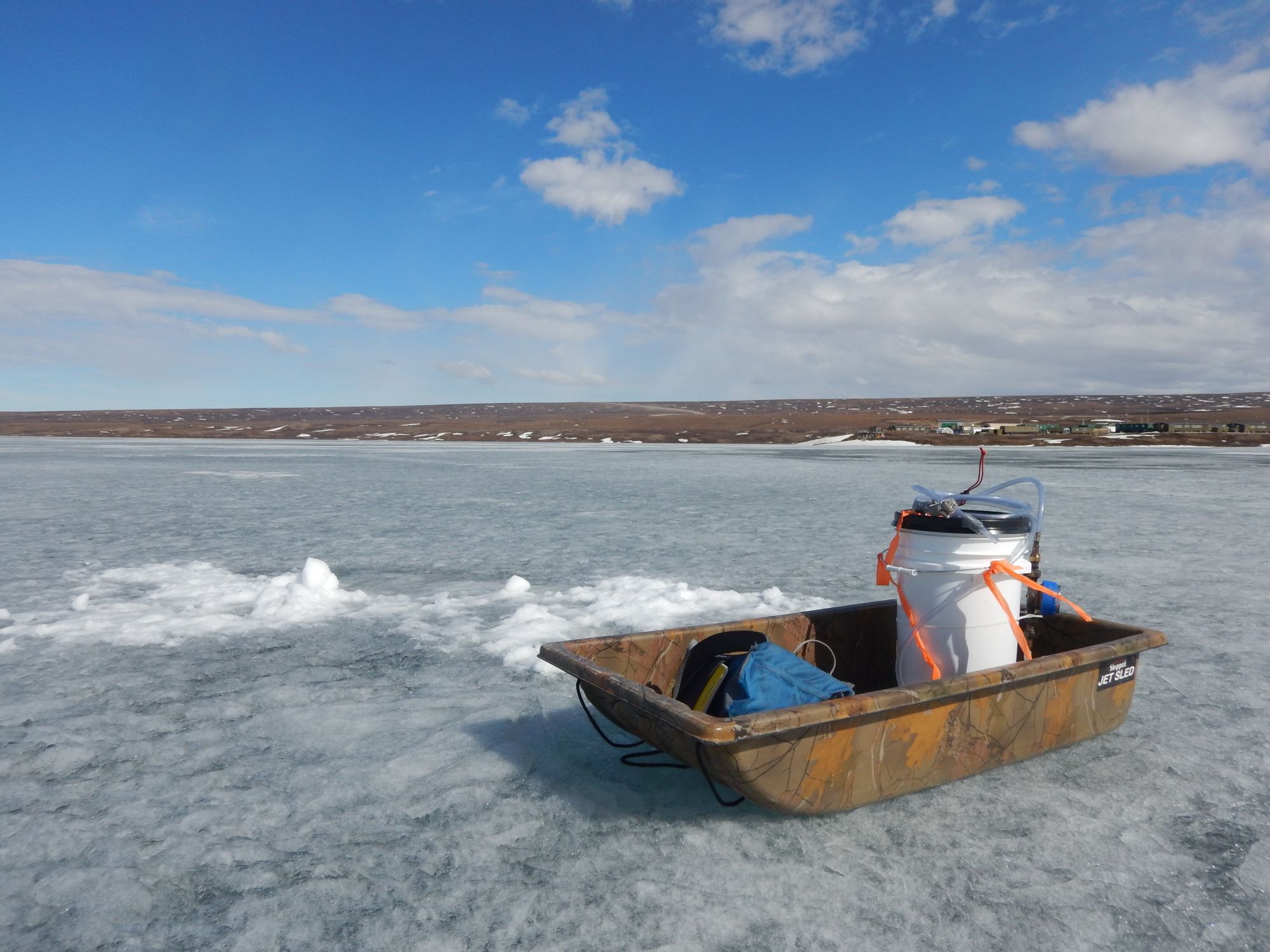
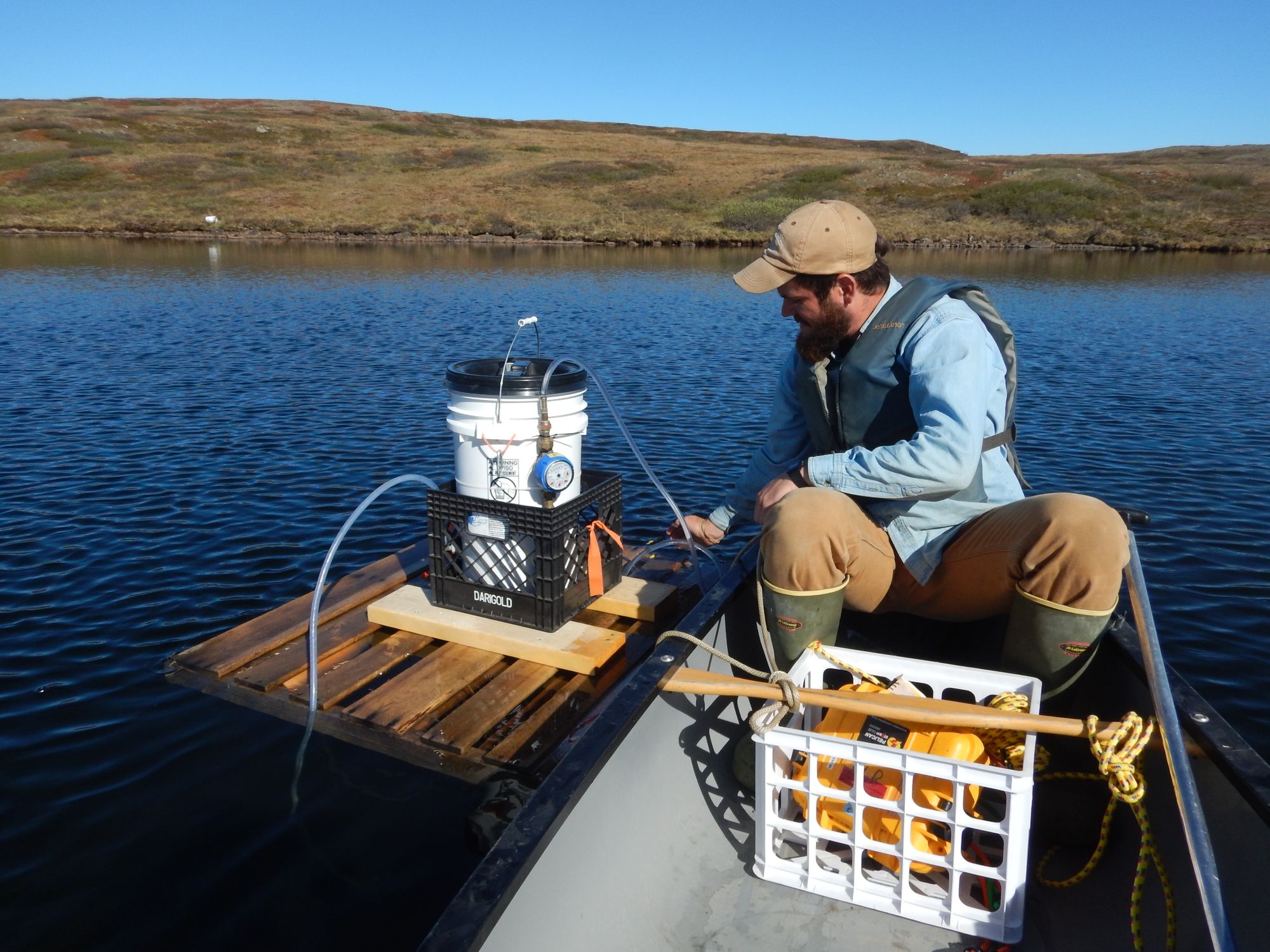
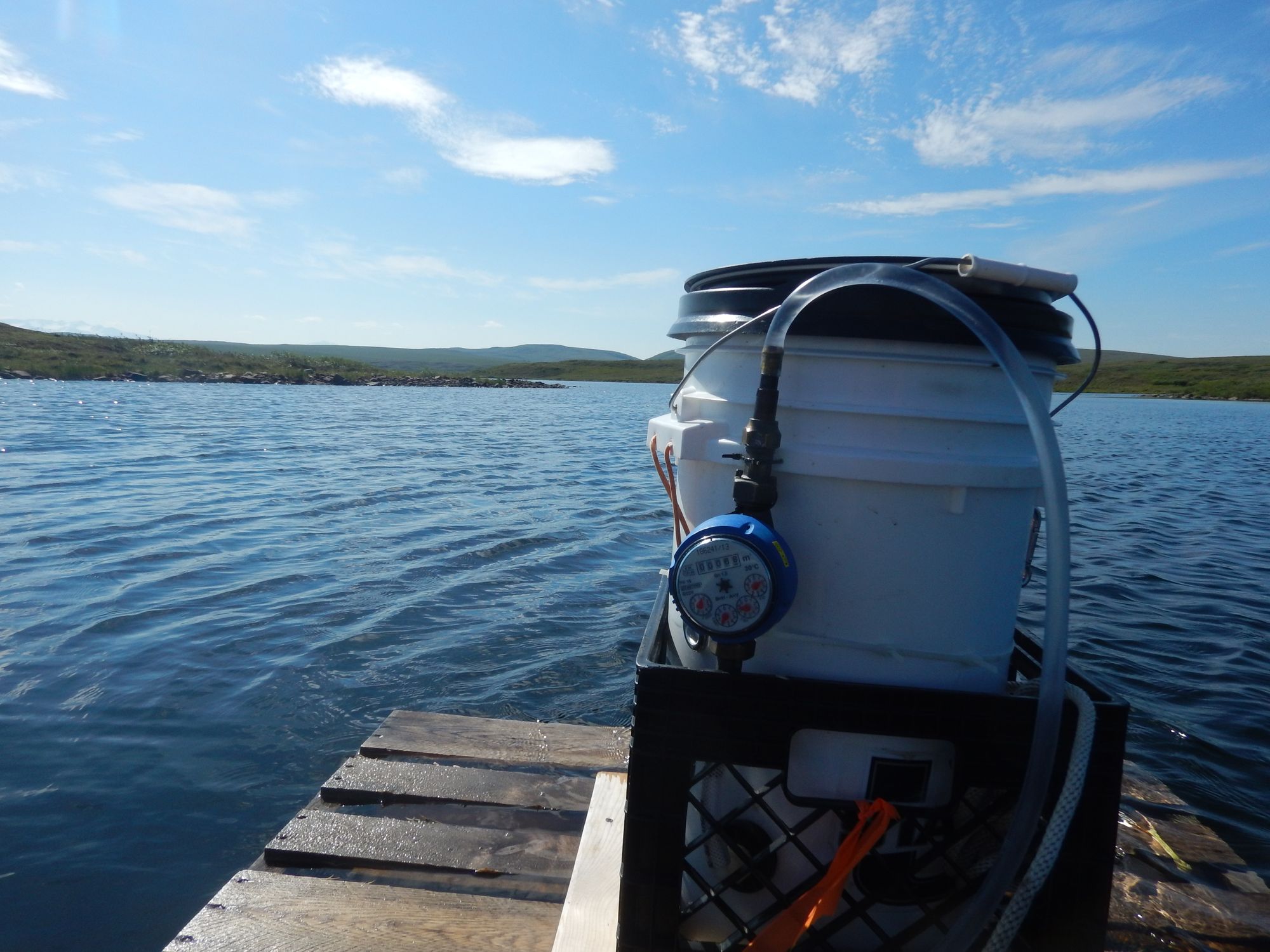
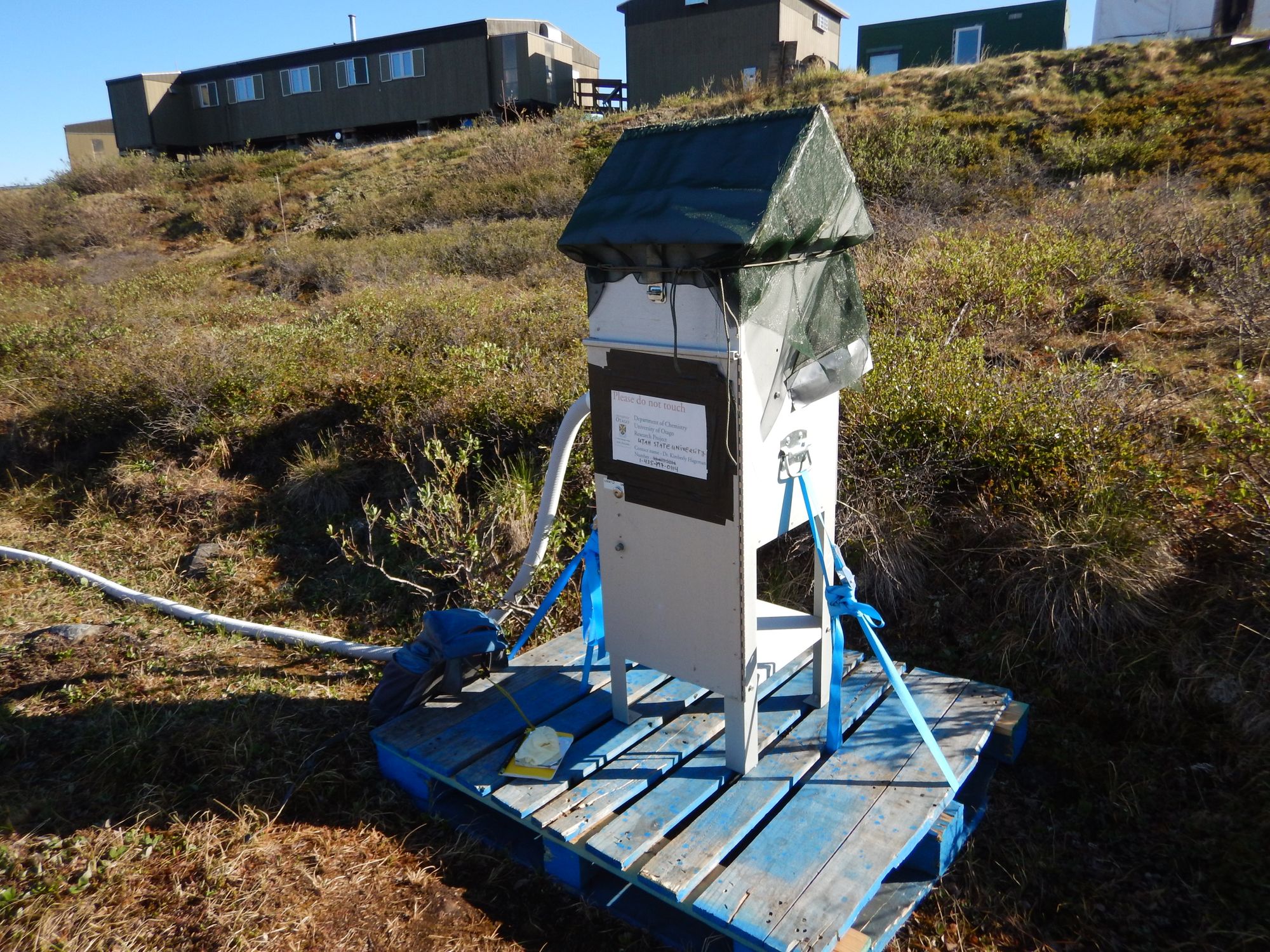
🔶Organic Contaminants in Great Salt Lake
BACKGROUND
Utah’s Great Salt Lake, like many saline lakes worldwide, is declining in surface area as upstream water use increases in its watershed. Due to the fact that they have no outflow, and the high levels of upstream urban and agricultural activity, the Great Salt Lake is also particularly susceptible to accumulation of contaminants in its sediments. As lake levels decline, large areas of these sediments are now becoming dry lake bed that then become a source of airborne dust.
WHAT WE DO
We care about the potential health risks of the nearby communities through inhalation exposure to the dust, in particularly, of organic contaminants in the dust. Therefore, we and our collaborators collect sediment and dust samples from the dry lake bed and measure a variety of harmful compounds that may be present including cyanotoxins, heavy metals, and organic contaminants.
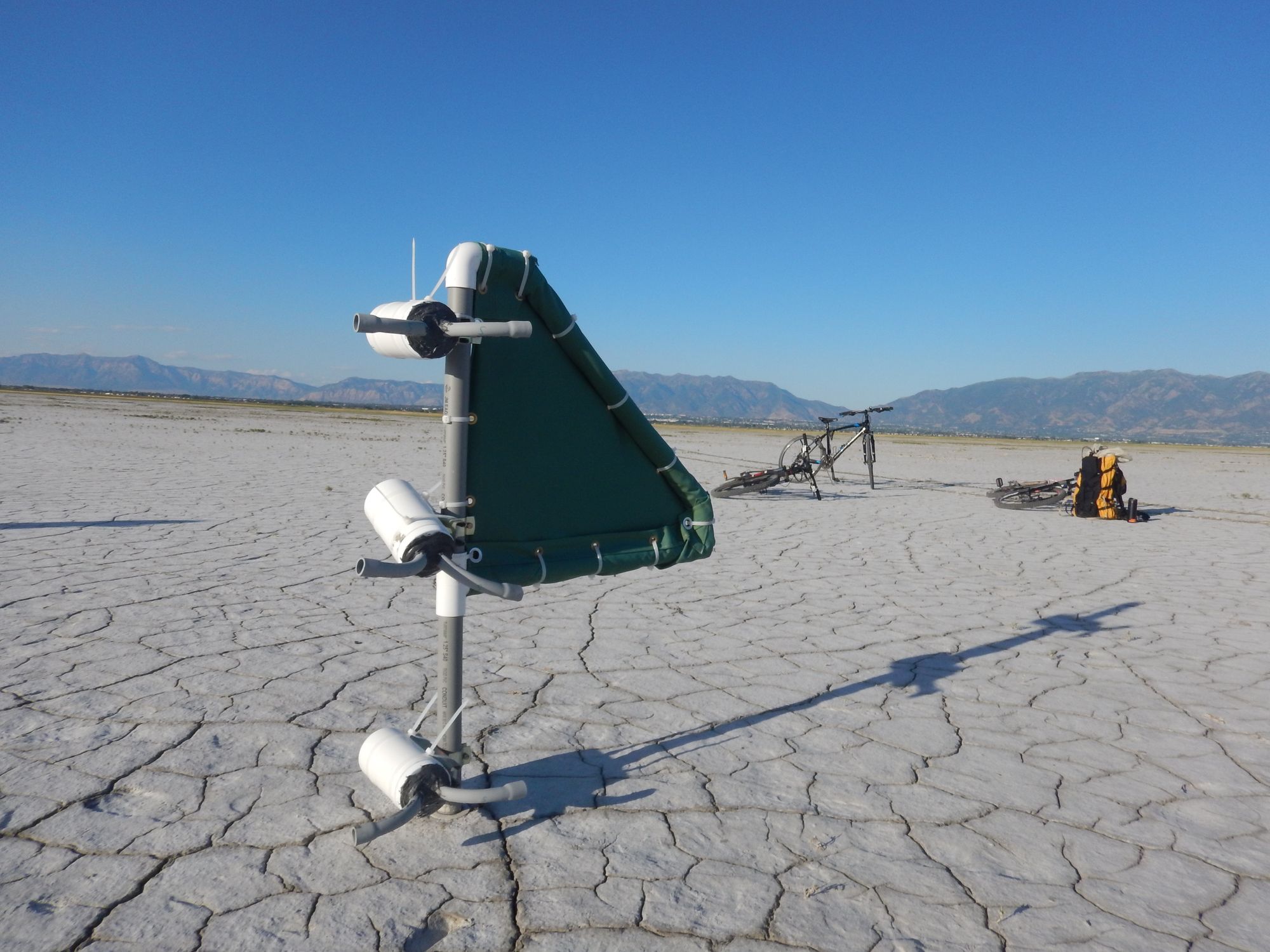
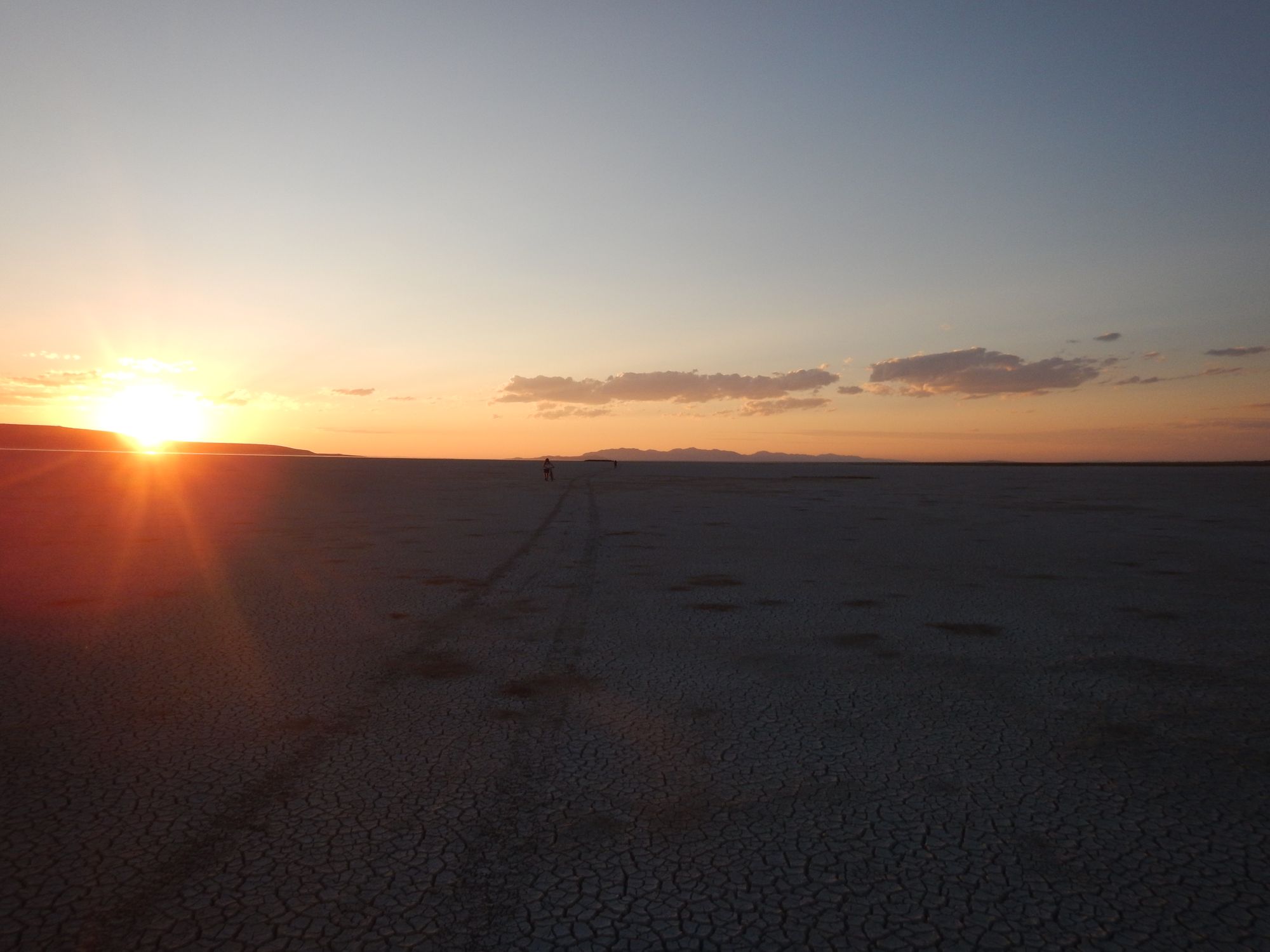
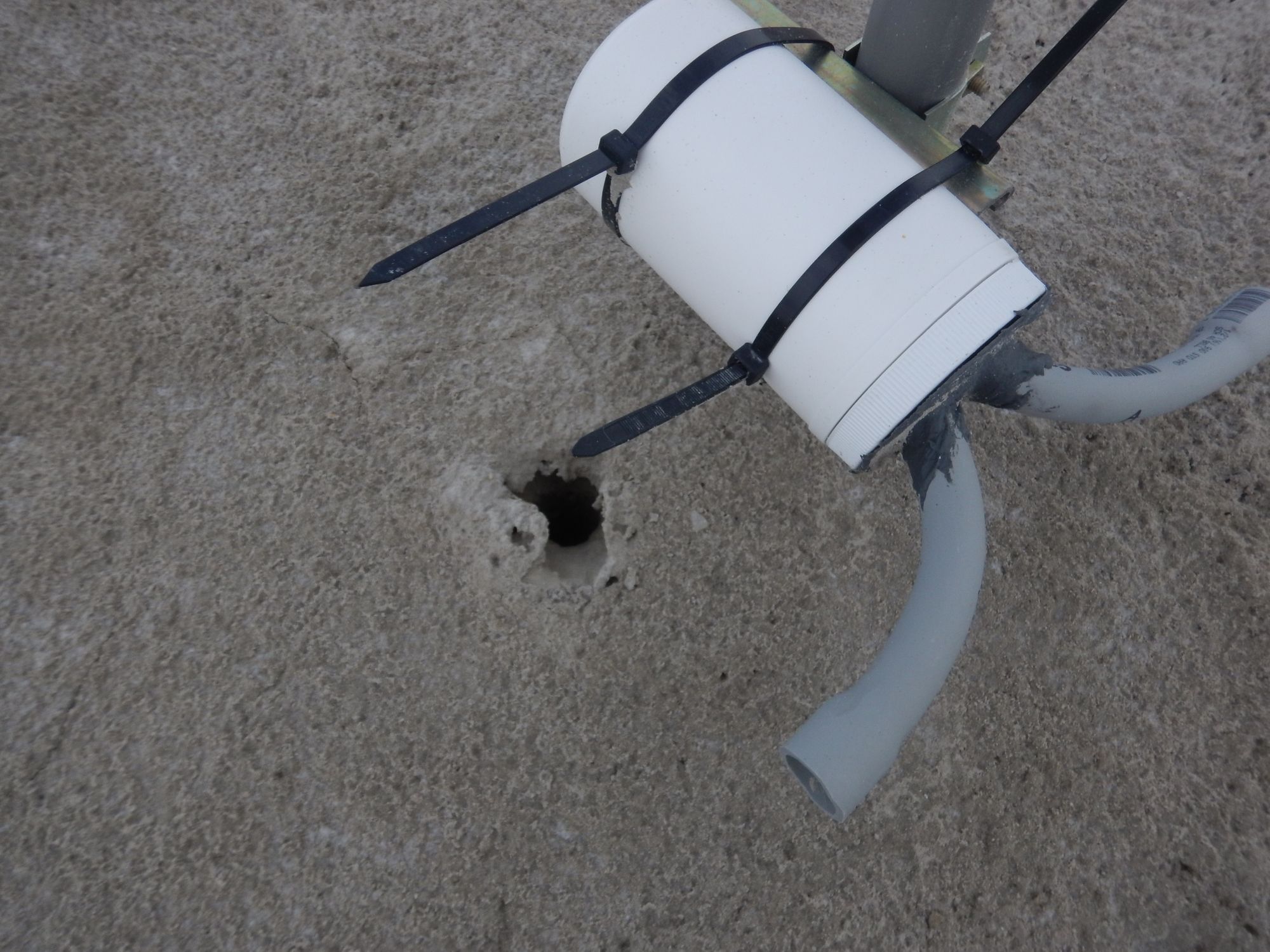
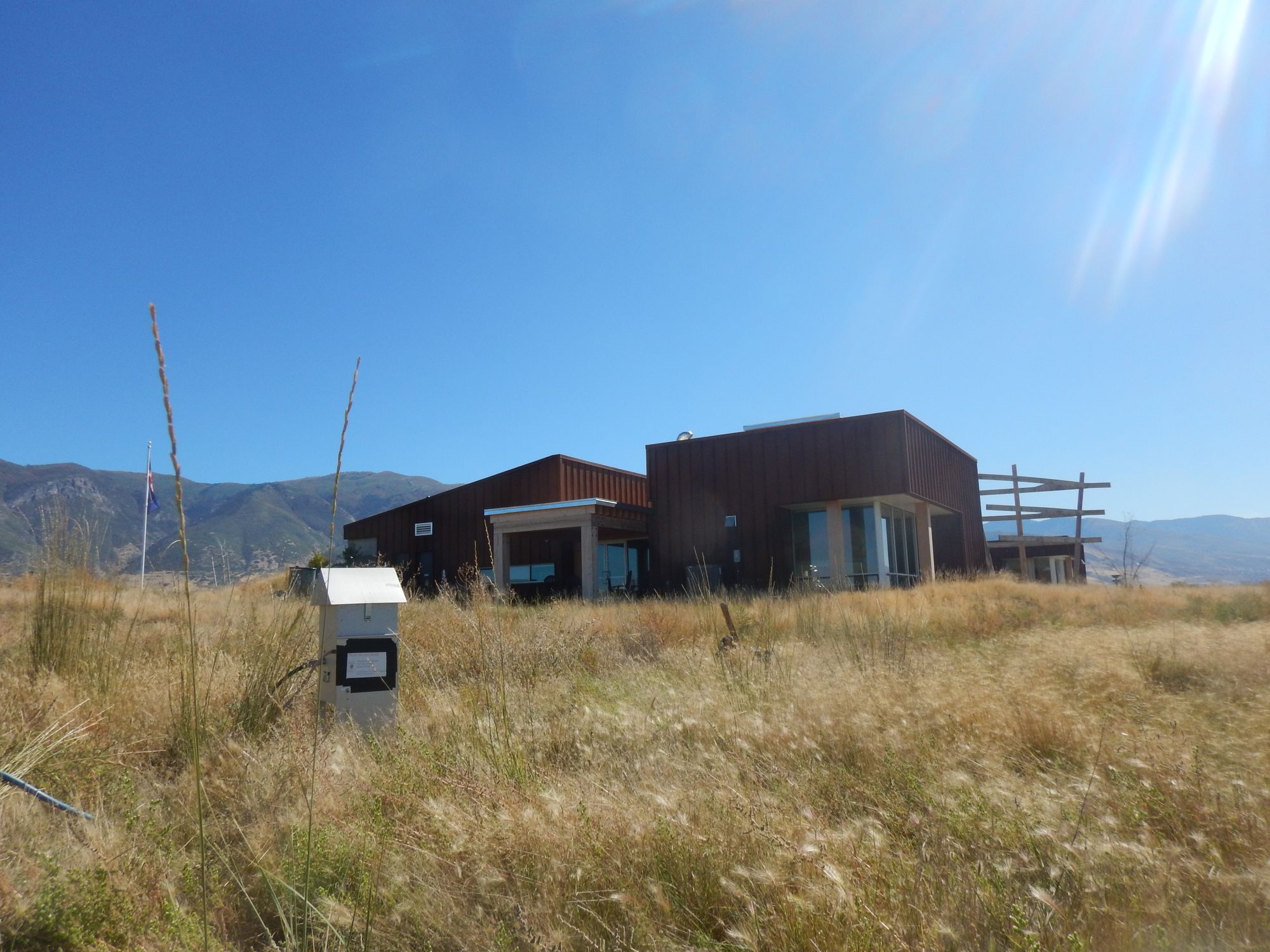
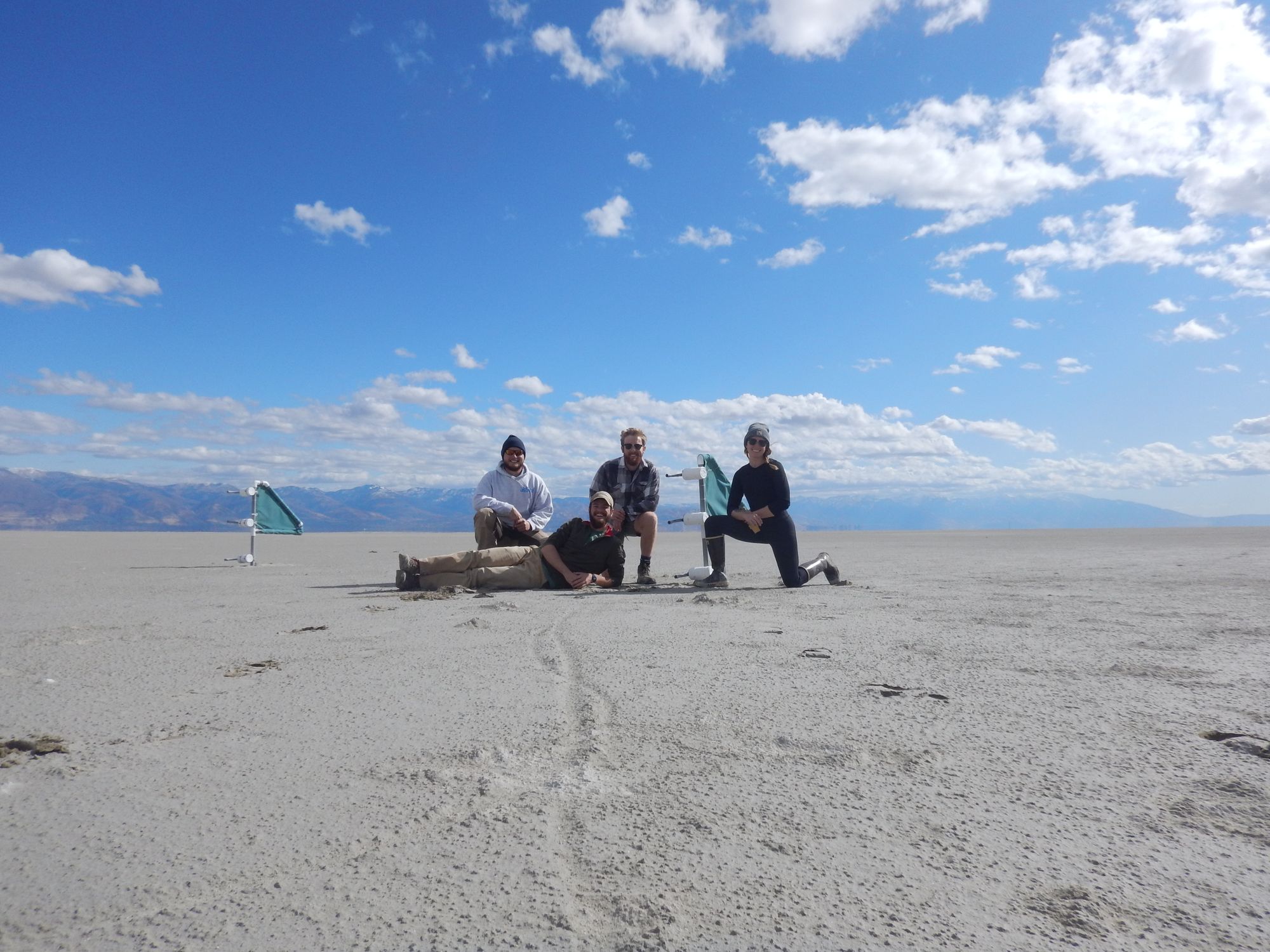